QUKIT
State of the art and beyond
The QuKiT approach to quantum computing brings together several disciplines such as materials growth, topological superconductivity, superconducting qubits and hybrid cQED.
Research areas
Research areas
Material Development
Hybrid qubits tailored wafers
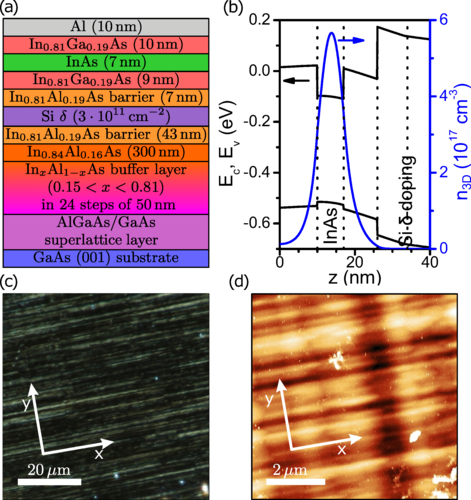
The Kitaev Chain
Enhanced protection
Majorana Bound states (MBS) are degenerate, non-local states of a one-dimensional system with properties that are robust against any local perturbation. Qubit platforms utilizing these MBS are expected to exhibit enhanced protection of topological origin against dielectric loss and magnetic noise.
MBS have been experimentally realised in superconductor – semiconductor quantum dots based nano devices referred to as ‘Kitaev Chains’. The QuKit project will focus extensively on experimentally realizing short Kitaev chains in optimized III-V 2DEG platforms and extending the Kitaev-chain devices to implement the Kitmon. This would involve improving the existing nano-fabrication techniques for hybrid semiconductor-superconductor heterostructures, simulation and development of readout and tune-up techniques for the extended Kitaev-chain system.
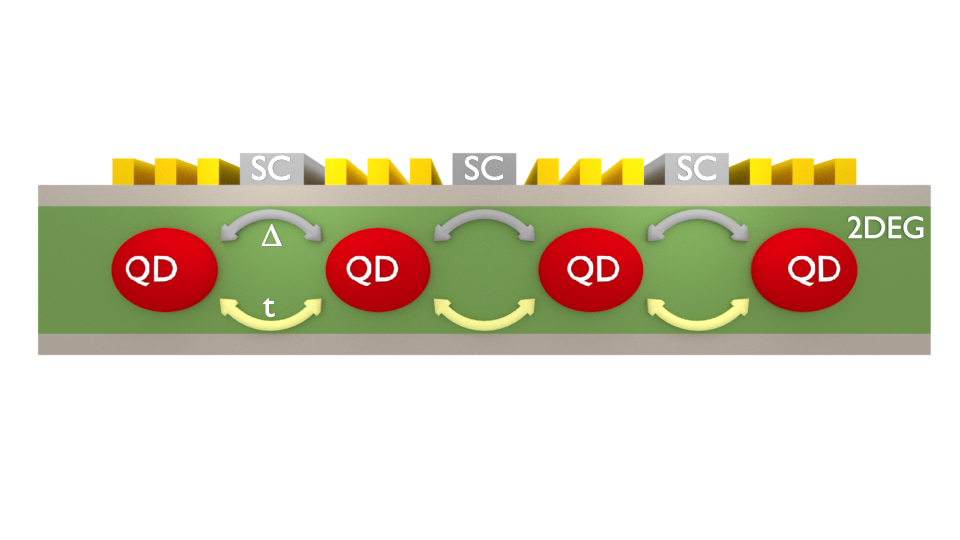
Kitmon
Novel hybrid qubit
This is a qubit that explicitly makes use of near-degenerate modes of short Kitaev chains. Specifically, by connecting two chains of two sites each with a tunnel coupling (four quantum dots(QDs) in total, the minimal number of dots needed to create a qubit), an effective Josephson junction forms with an energy difference that is 4π periodic in the superconducting phase difference between the two Kitaev chains. This 4π periodic potential effectively creates a qubit subspace based on the even/odd parity between the two arrays.
Kitmon is expected to show improvements in qubit lifetimes and gate fidelity compared to current mainstream approaches, and would serve as a proof of principle for the QuKiT technology.
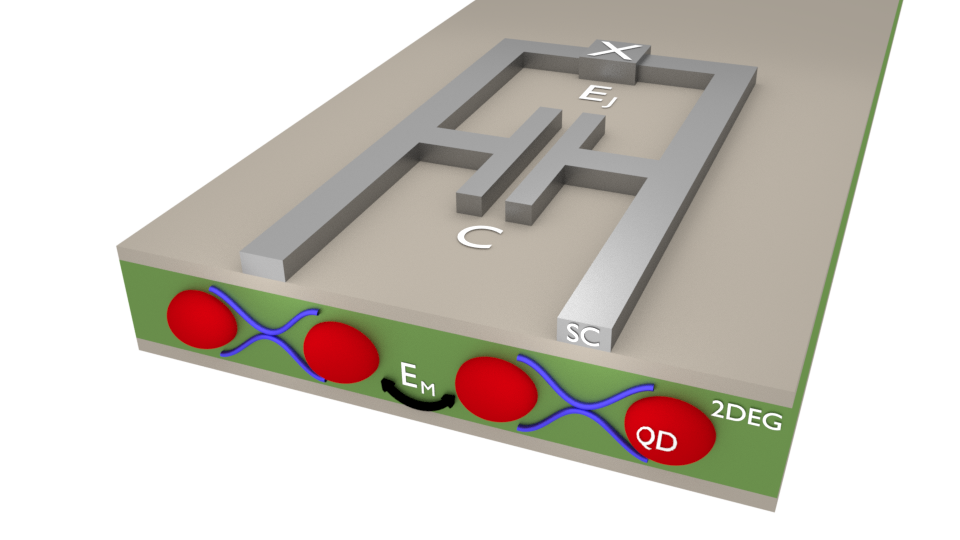
Hybrid cQED
Optimal circuit for kitmon
This emerging field explores new device functionality and machanism that replaces conventional tunnelling Josephson junctions (JJs) with semiconductor-superconductor hybrid JJs, and embeds quantum dots(QDs) defined on various materials in superconducting circuits. Base on the design of Kitmon, this is a vital subject that must be well understood, optimized, and deeply exported .
Recently, a collaborative effort between the lab of Christian Kraglund Andersen and the theory group of Ramon Aguado has shown for the first time how the Andreev levels in a JJ comprising a spin-½ QD can be used to realize a superconducting spin qubit.
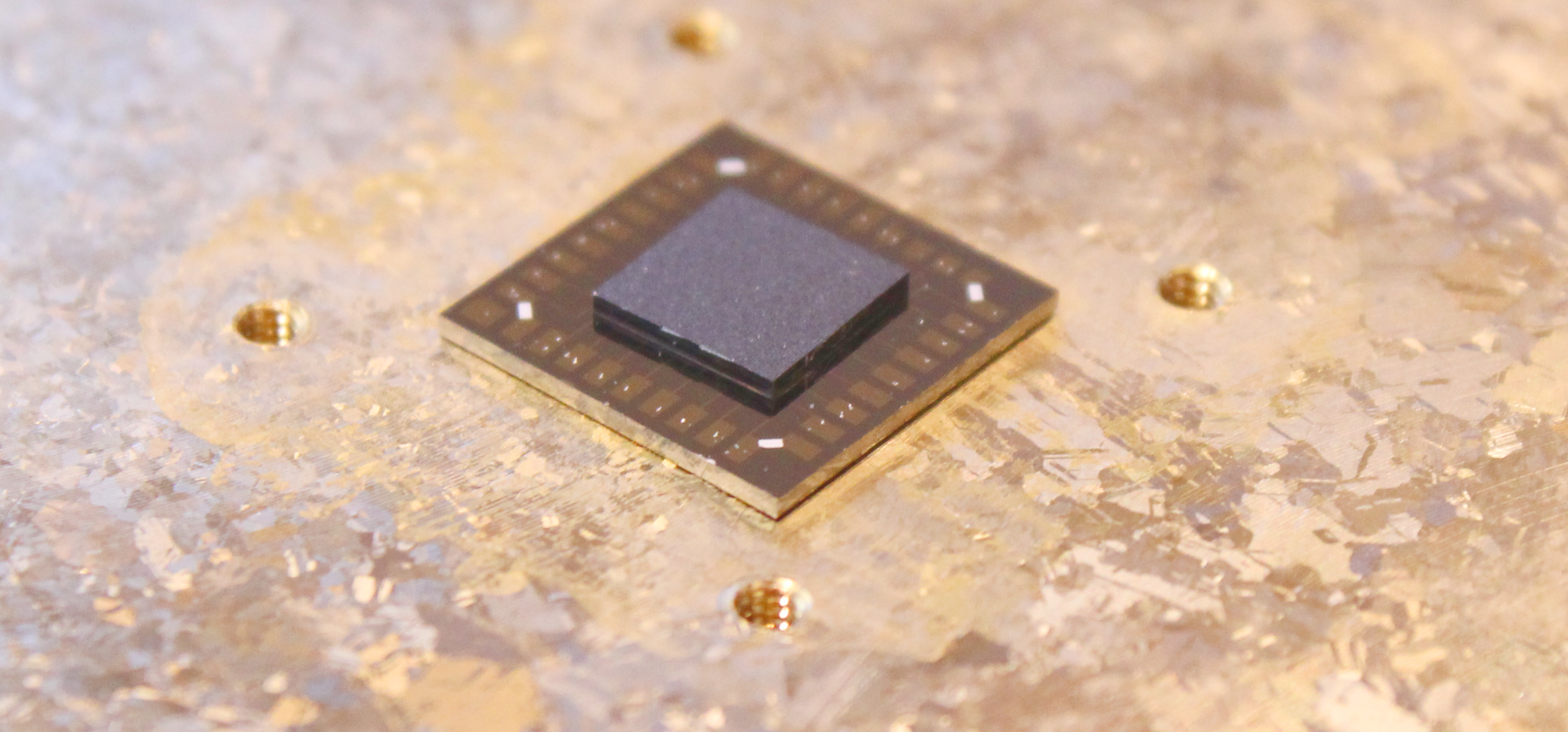
Control system engineering
Semiconductor-superconductor hybrid system manipulation
Numerous control signals must be implemented to realize Kitmon. For example, the quantum dots(QDs) of the Kitaev chain are defined with electrostatic gates on a 2DEG. Moreover, the QD tunnel coupling, superconducting coupling, and tunnel coupling between the Kitaev chains must be fine tuned with certain control signals. In addition, the Josephson junction of a Kitmon will be defined as an SNS junction, which means a control signal is also needed to implement gate-tunable Josephson energy (EJ).
All control signals are routed from milli-Kelvin to room-temperature using best practices of the field and the signals are produced by room-temperature electronics. We will rely on commercial solutions for such a system, provided by industry partner Orange Quantum Systems B.V. They have recently launched a complete control system for conventional transmon qubits, and this will serve as a starting point to develop a product specifically designed for the Kitmon system.
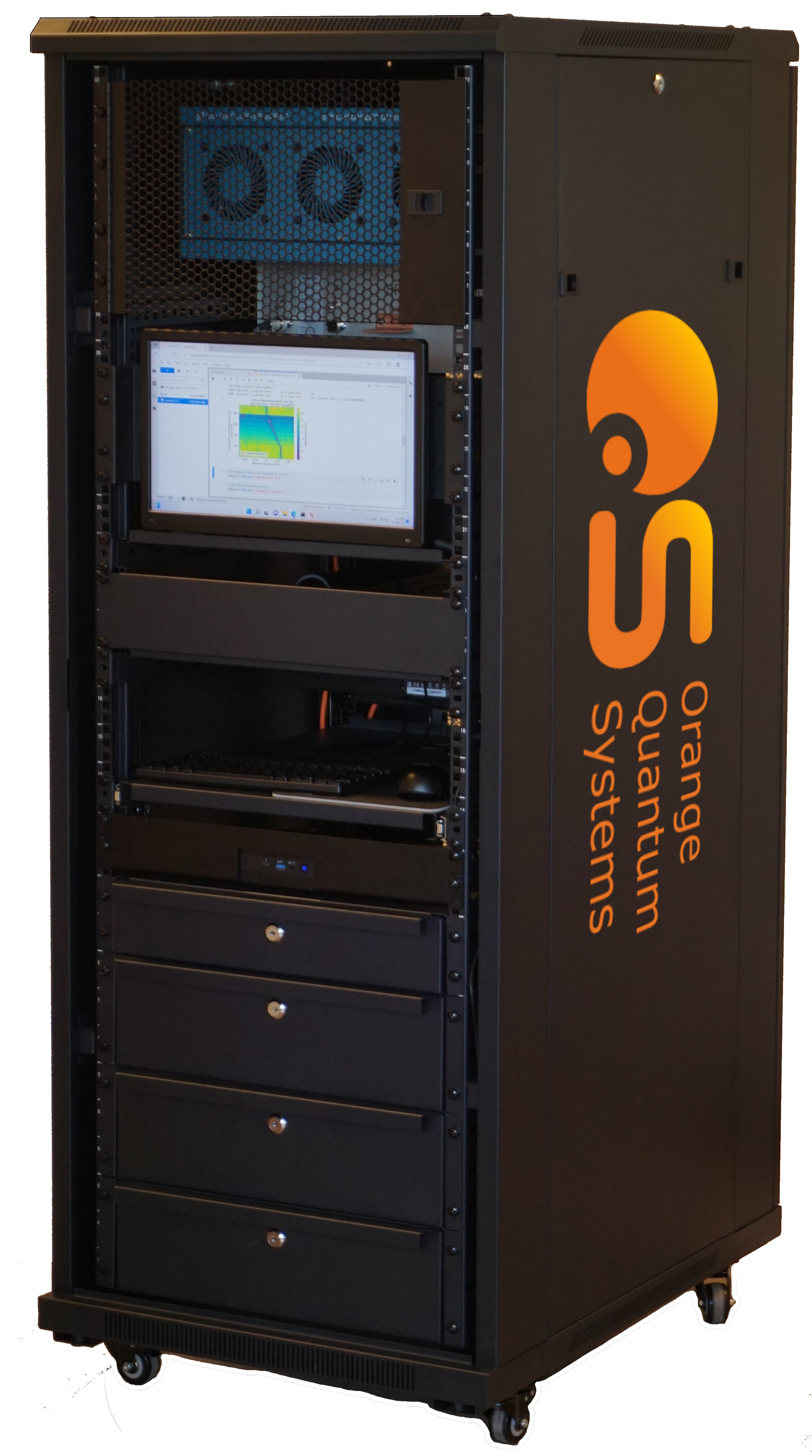